Model
Introduction to Version 5
Robert L. Walko, University of Miami
The Ocean-Land-Atmosphere Model (OLAM) is a global non-hydrostatic weather and climate simulation model that has been developed and applied since the early 2000s (Walko and Avissar 2008a, b, 2011). It is an outgrowth of the Regional Atmospheric Modeling System (RAMS; Cotton et al., 2003), a widely-used research model that has been applied in numerous investigations of mesoscale and cloud-scale phenomena for nearly three decades. OLAM was originally constructed as a global version of RAMS that combined the advantages of regional and global models by utilizing a variable-resolution global gridding system with two-way interaction across scales. Recent versions of OLAM accomplish this with a seamless unstructured grid consisting of hexagonal cells, along with a few pentagons and heptagons (Fig 1). This unification of multiple modeling scales within a single framework enables high-resolution modeling in selected geographic regions of interest while avoiding the problems and inherent limitations of applying lateral boundary conditions in limited-area models. Similarly, low resolution simulations that have a global emphasis can take advantage of selectively higher resolution of important features, such as major mountain ranges (Figure 2). Local mesh refinements are constructed as resolution-doubling steps, but the onset of each step is gradual over a distance of 4 grid cells; this helps to minimize any reflections, in contrast to the more abrupt resolution transitions of conventional grid nesting schemes.
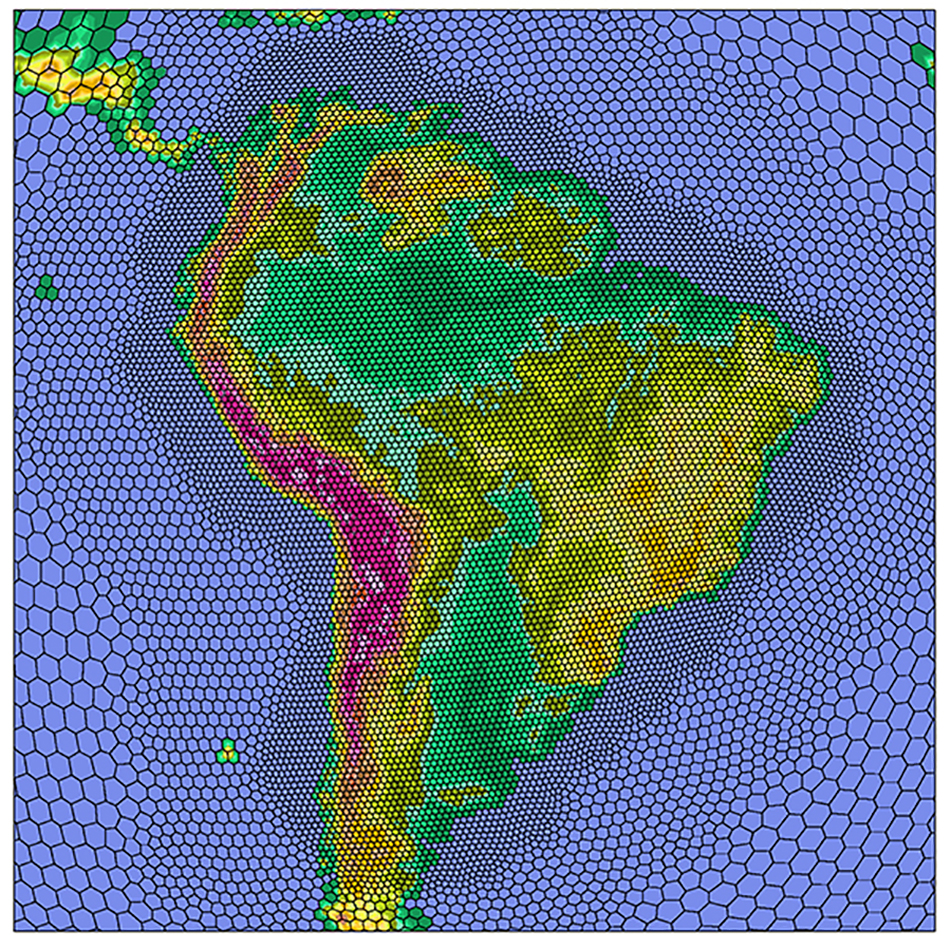
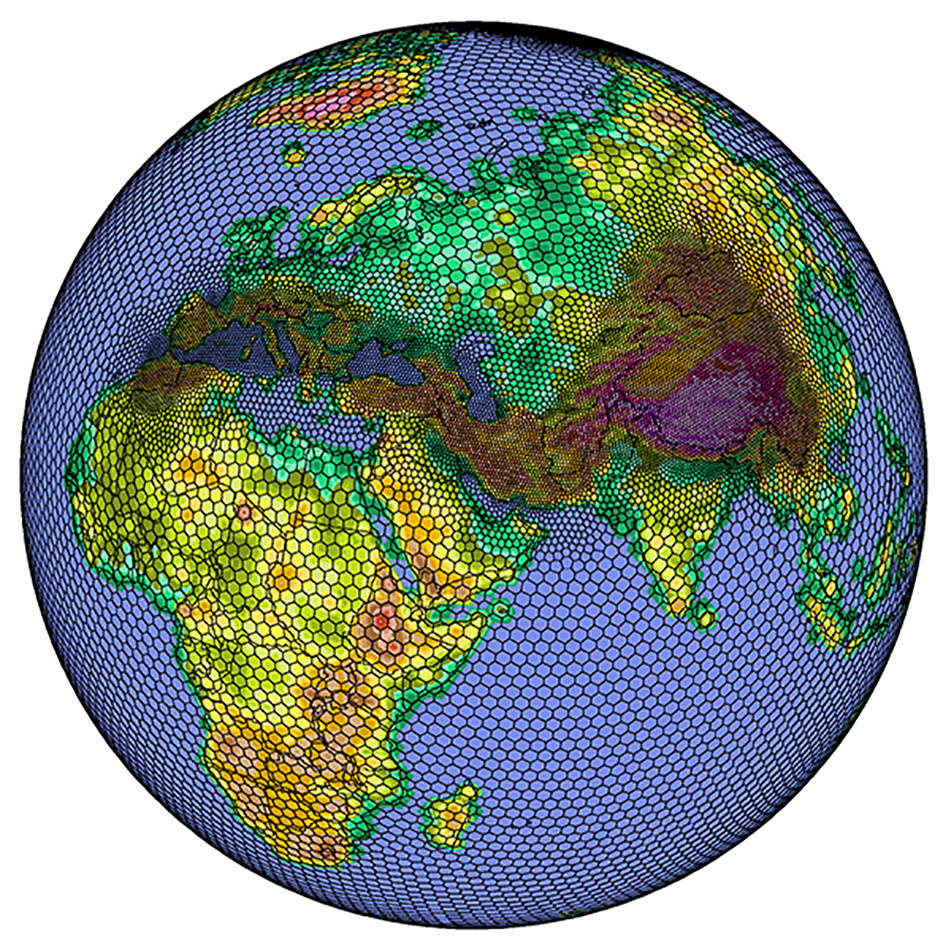
A unique feature of OLAM’s grid is that it does not employ a terrain-following transformation but instead uses horizontal grid levels that intersect topography (Figure 3). Cut grid cells with reduced volumes and face areas are used at the intersections to represent partial submergence below the surface. In combination with a finite-volume discretization of the dynamic core equations, this formulation satisfies the kinematic boundary condition at the surface and preserves mass, momentum, and energy conservation properties of the governing equations. Use of horizontal grid levels avoids the well-documented errors that terrain-following coordinates introduce in horizontal gradient computations, especially of pressure, and also avoids problematic grid imprinting on the model solution above complex topography. OLAM solves the full compressible, non-hydrostatic, deep-atmosphere formulation of the Navier-Stokes equations in finite-volume conservation form. For numerical efficiency, horizontal and vertical acoustic modes are time split, and vertical acoustic modes are solved implicitly.
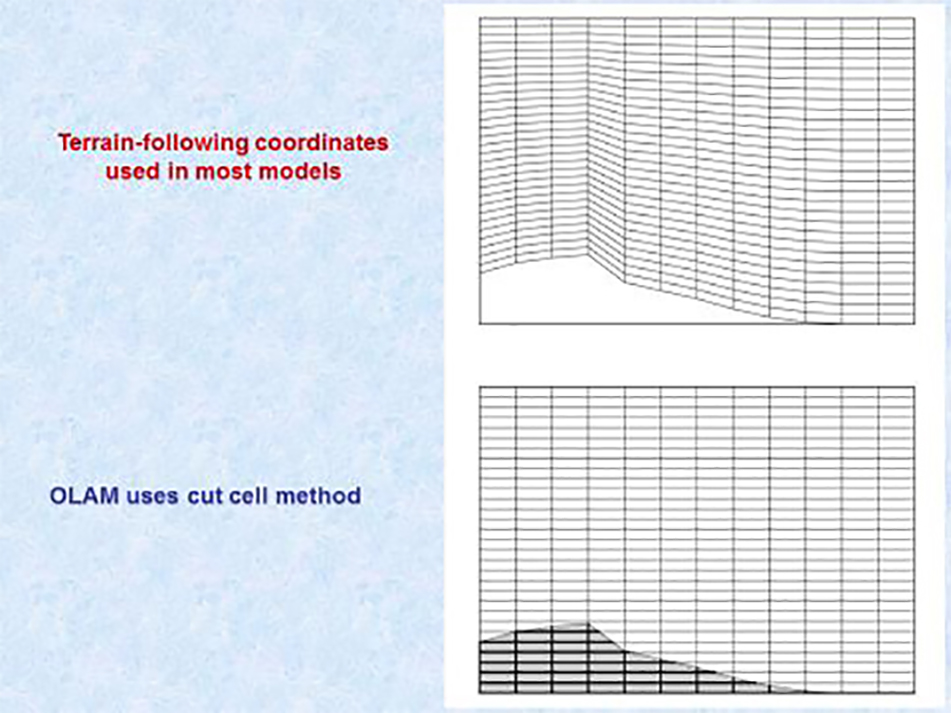
While OLAM has evolved independently of its RAMS predecessor, both models continue to share two-moment bulk microphysics parameterization (Walko et al., 1995, 2000a; Meyers et al., 1997 Cotton et al. 2003), which represents atmospheric hydrometeors in several different liquid, ice, and hybrid sizes and forms and is one of the most advanced schemes in existence. The parameterization employs several bin-emulating computations, where processes are pre-computed for multiple bins of hydrometeor sizes and summed over bins to obtain a more accurate result than possible from the bulk formulas. Results from the pre-computations are stored in lookup tables where they are efficiently accessed and applied during model runs. Recent upgrades to the microphysics include explicit representation of aerosols and their impact on liquid and ice nucleation (including aerosol size, concentration, and chemistry). Prognosis of multiple aerosol species in OLAM includes nucleation and precipitation scavenging by hydrometeors, anthropogenic sources, and natural sources from vegetation, sea spray, wind-driven dust, and photo-chemistry. The CMAQ and GEOS-Chem chemistry models have been interfaced to OLAM and represent additional aerosol sources and sinks. Radiative properties of hydrometeors are based in part on Key et al. (2002) and interface to the Rapid Radiative Transfer Model (RRTMg) (Mlawer et al, 1997; Clough et al., 2005) which evaluates radiative fluxes, scattering, absorption, and emission between the atmospheric, land, and surface water components of OLAM.
The other principal component of RAMS that is used in OLAM is the Land-Ecosystem-Atmosphere Feedback (LEAF) model (Walko et al. 2000b), a Soil-Vegetation-Atmosphere Transfer (SVAT) model that represents energy and mass conservation and exchange at the lower boundary of the atmosphere. In its most basic form, LEAF is a column model consisting of multiple soil/bedrock layers, vegetation, a vegetation canopy air layer, and “surface water” layers to accommodate snow cover or standing water that is either a wetland or has not had time to run off or infiltrate during or following a precipitation event. Water and energy are stored in each component and exchanged between components and the atmosphere, subject to conservation laws, heat conduction and Richard’s equation in the soil, turbulent transfer relationships in the canopy and atmosphere, a stomatal conductance formulation, and radiative transfer. Latent heat energy of freezing and melting are accounted for in all components of LEAF. For a more complex treatment of vegetation, the Ecosystem Demography Version 2 Model (ED2) developed by Medvigy et al (2008) provides an optional representation of hydrology, land-surface biophysics, vegetation dynamics and soil carbon and nitrogen biogeochemistry. The fast timescale fluxes of carbon, water, and energy between the land surface and the atmosphere are captured using leaf photosynthesis and soil decomposition modules coupled to a multi-leaf layer and multi-soil layer biophysical scheme. Like its predecessor ED (Moorcroft et al 2001), ED2 captures sub-grid scale biotic heterogeneity arising from disturbance events using a system of size- and age-structured partial differential equations that closely approximate the ensemble mean behavior of a corresponding individual-based stochastic gap model. With this system of equations, ED2 is also able to incorporate the impacts of subgrid-scale disturbances on the structure and function of the land surface within each grid cell. This includes natural disturbances such as wind-throw and natural fires, and anthropogenic disturbances such as land conversion and fire activity (e.g., Hurtt et al 2002).
OLAM generates an unstructured surface grid for land, lake, and ocean areas that, like the atmosphere grid, can be locally refined over any geographic region(s) and to any degree desired. Parameters for the surface grid may be chosen independently of those in the atmosphere grid, enabling local mesh refinement to be tailored for different needs, such as higher ocean resolution in coastal areas or higher land resolution in regions of complex topography. The ocean grid structure in OLAM currently permits two options, one in which sea surface temperature is specified from time-dependent observations, and the other in which sea temperature, salinity, momentum, and other properties are predicted at the surface and deeper levels from a one-dimensional turbulent mixing formulation. For the latter option, a set of routines has been adapted from the General Ocean Transport Model (GOTM) (Burchard et al., 2006) (www.gotm.net). The 3D HYCOM ocean model was previously coupled to OLAM, but it is not currently supported, pending the implementation of a 3D ocean model that permits horizontally-variable resolution.
References
Burchard, H., K. Bolding, W. Kühn, A. Meister, T. Neumann, and L. Umlauf, 2006: Description of a flexible and extendable physical-biogeochemical model system for the water column, Journal of Marine Systems, 61, 180-211.
Clough, S. A., M. W. Shephard, E. J. Mlawer, J. S. Delamere, M. J. Iacono, K. Cady-Pereira, S. Boukabara, and P. D. Brown, 2005: Atmospheric radiative transfer
modeling: a summary of the AER codes, Short Communication, J. Quant. Spectrosc. Radiat. Transfer, 91, 233-244.
Cotton, W.R., R.A. Pielke, Sr., R.L. Walko, G.E. Liston, C.J. Tremback, H. Jiang, R.L. McAnelly, J.Y. Harrington, M.E. Nicholls, G.G. Carrió.P. McFadden, 2003: RAMS 2001: Current status and future directions. Meteor. Atmos Physics, 82, 5-29.
Hurtt, G.C., S.W. Pacala, P.R. Moorcroft, J. Caspersen, E. Shevliakova, R.A. Houghton and B. Moore,
2002. Projecting the future of the U. S. Carbon sink, Proc. Nat. Acad. Sci., 99, 1389-1394.
Key, J.R., P. Yang, A.B Bryan, and S.L.Nasiri, 2002: Parameterization of shortwave ice cloud optical properties for various particle habits. J. of Geophysical Res., 107 (D13), 10.1029/2001JD000742.
Medvigy, D., S.C. Wofsy, J.W. Munger, D.Y. Hollinger, and P.R. Moorcroft, 2009. Mechanistic scaling
of ecosystem function and dynamics in space and time: the Ecosystem Demography model version 2.
J. Geophys. Res., 114, G01002.
Meyers, M.P., R.L. Walko, J.Y. Harrington, and W.R. Cotton, 1997: New RAMS cloud microphysics prameterization. Part II: The two-moment scheme. Atmos. Res., 45, 3-39.
Mlawer, E.J., S.J. Taubman, P.D. Brown, M.J. Iacono and S.A. Clough, 1997: RRTM, a validated correlated-k model for the longwave. J. Geophys. Res., 102, 16,663-16,682.
Moorcroft, P.R., G.C. Hurtt and S. Pacala. 2001. A Method for Scaling Vegetation Dynamics: The
Ecosystem Demography Model (ED). Ecological Monographs. 71, 557-586.
Walko, R.L, W.R. Cotton, J.L. Harrington, M.P. Meyers, 1995: New RAMS cloud microphysics parameterization. Part I: The single-moment scheme. Atmos. Res., 38, 29-62.
Walko, R.L., W.R. Cotton, G. Feingold, B. Stevens, 2000a: Efficient computation of vapor and heat diffusion between hydrometeors in a numerical model. Atmos. Res., 53, 171-183.
Walko, R.L., L.E. Band, J. Baron, T.G.F. Kittel, R. Lammers, T.J. Lee, D. Ojima, R.A. Pielke, C. Taylor, C. Tague, C.J. Tremback, and P.L. Vidale, 2000b: Coupled atmosphere-biophysics-hydrology models for environmental modeling. J. Appl. Meteor., 39, 931-944.
Walko, R. and R. Avissar, 2008a. The Ocean-Land-Atmosphere Model (OLAM). Part I: Shallow Water Tests. Mon. Wea. Rev., 136, 4033-4044.
Walko, R. and R. Avissar, 2008b. The Ocean-Land-Atmosphere Model (OLAM). Part II: Formulation and Tests of the Nonhydrostatic Dynamic Core. Mon. Wea. Rev., 136, 4045-4062.
Walko, R. L., and R. Avissar, 2011: A direct method for constructing refined regions in unstructured conforming triangular-hexagonal computational grids: Application to OLAM. Mon. Wea. Rev., 139, 3923-3937.